AMET University
AMET University | |
---|---|
![]() | |
Motto | Steering Quality Education |
Established | 1992 |
Type | Maritime, Deemed |
Chairman | J. Ramachandran |
Chancellor | D. T. Joseph, IAS (Retd) |
Vice-Chancellor | Capt. S. Bhardwaj |
Students | 2500+ |
Location | Chennai, Tamil Nadu, India |
Affiliations | All India Council for Technical Education(AICTE), International Association of Maritime Universities (IAMU) Royal Institute of Naval Architecture (RINA), University Grant Commission (UGC), Directorate General of shipping |
Website | www.ametuniv.ac.in |
AMET University, formerly the Academy of Maritime Education (AMET), is an educational institute in India dedicated for maritime and maritime related education and training. AMET University is Situated on the outskirts of the city of Chennai . It is an autonomous engineering and technology oriented institute of higher education in India. It is a Deemed University under section 3 of the University Grants Commission Act, 1956. It is India's first maritime university that runs only a few number of courses. But has programs even leading up to PhD degree.
History
It was founded in 1992 as the "Academy of Maritime Education and Training" which was dedicated to maritime studies. Within the first few years this academy became one of the best in maritime and related education. In the early years AMET was able to tie up with many international maritime colleges like South Tyneside College, Hull College, Glasgow College of Nautical Sciences etc. During the early stages AMET was affiliated to Birla Institute of Technology Mesra( BIT Ranchi). It was BIT Ranchi who gave certificates to marine engineering, nautical science, GME AND, management students. In 2007 AMET was given university status by Ministry of HRD, India under de-novo category. NAVAL ARCHITECTURE Dept. was founded in 2007 , making the University one among the 5 institutes having this Dept.

The Jewel of AMET, a model ship located in the AMET campus
Administration
AMET functions under A Board of Advisors comprising representatives of various shipping sectors including Govt. and Private ones. J. Ramachandran is the founder chairman of the university.. He is also the founder chairman of a social welfare organisation called NAESEY. He also head's Saraswathi Institute of Medical Sciences. D. T. Joseph, IAS (Retd) is the chancellor of the University . A former Director General Of Shipping as well as Secretary Shipping, Govt. of India. Capt. S Bhardwaj is presently the vice chancellor of the university.
Board of Advisors
AMET has an international board of advisors. It includes :
- Ib Fruergaard, Managing Director, Mari-time Solutions, (Singapore) Pte Ltd.
- G. Ramaswamy, Chief Executive Officer, Sea Team Management (India) Pvt.Ltd, Chennai (Frontline Group, Norway).
- Bjorgulf Haukelid,Vice President, Det Norske Veritas, Norway.
- Satoru Kuboshima, President, K-Line Ship Management. Japan
- Baptista Sumbe, President & CEO, Sonangol USA Company, Houston, Texas, USA .
- Rajaish Bajpaee, COO, Bernhard Schulte Shipmanagement, Hong Kong
- Swapan Das Sarma, Director, Teledata Marine Solutions, USA .
- Robert J. Bel, Chief Executive Officer, Archomai Group, U.K (Also on the advisory board of The University of Hull, Logistics Institute, Hull)
- Bjarne Foldager, A.P.Moller Singapore (Pte) Ltd, Singapore .
- L. R. Chary, (FIMarE) Former Executive Director, Shipping Corporation of India Ltd.
Admissions
Admissions for AMET are based on All India level online exams in respective fields.Preliminary round of entrance examinations are done online ,and the next round will be held after shortlisting the candidates at the university campus .
Campus Facilities
- Hi speed 24 hour Wi-fi connection through out the campus including hostels
- Corporation Bank ATM
- Basketball Court
- Volley ball and Kabaddi court
- Mini- olympic Swimming pool
- Indoor games Hall
- Gymnasium
- Football court
- Unique Ship in Campus
- George T R Campell Library
- A.P Mollar Centrally Air Conditioned Auditorium
- Polaris Bridge Simulator from Kongsberg
Departments :
The major departments are:
- Naval architecture
- Nautical Science
- Marine engineering
- Harbour engineering
- Petroleum engineering
- Maritime Management
- Electrical engineering
- Bio-Technology
Naval architecture :

Tahitian Princess in Tórshavn, Faroe Islands, August 2009
Naval architecture is an engineering discipline dealing with the design, construction, maintenance and operation of marine vessels and structures. Naval architecture involves basic and applied research, design, development, design evaluation and calculations during all stages of the life of a marine vehicle. Preliminary design of the vessel, its detailed design, construction, trials, operation and maintenance, launching and dry-docking are the main activities involved. Ship design calculations are also required for ships being modified (by means of conversion, rebuilding, modernization, or repair). Naval architecture also involves formulation of safety regulations and damage control rules and the approval and certification of ship designs to meet statutory and non-statutory requirements.
Main subjects
The word "vessel" includes every description of watercraft, including non-displacement craft, WIG craft and seaplanes, used or capable of being used as a means of transportation on water. The principal elements of naval architecture are:
- Hydrostatics
Body plan of a ship showing the hull form
- The conditions to which the vessel is subjected while at rest in water and its ability to remain afloat. This involves analyzing buoyancy (displacement) and other properties such as
- Trim - The longitudinal inclination of the vessel.
- Stability - The ability of a vessel to restore itself to an upright position after being inclined by wind, sea, or loading conditions.
- Hydrostatics
- Hydrodynamics
- Hydrodynamics concerns the flow of water around the ship's hull, bow, stern and over bodies such as propeller blades or rudder, or through thruster tunnels.
- Resistance - resistance towards motion in water primarily caused due to flow of water around the hull. Powering calculation is done based on this.
- Propulsion - to move the vessel through water using propellers, thrusters, water jets, sails etc. Engine types are mainly internal combustion. Some vessels are electrically powered using nuclear or solar energy.
- Ship motions - involves motions of the vessel in seaway and its responses in waves and wind.
- Controllability (manoeuvring) - involves controlling and maintaining position and direction of the vessel

- Structures
- Involves selection of material of construction, structural analysis of global and local strength of the vessel, vibration of the structural components and structural responses of the vessel during motions in seaway.
- Arrangements
- This involves concept design, layout and access, fire protection, allocation of spaces, ergonomics and capacity.
- Construction
- Construction depends on the material used. When steel or aluminium is used this involves welding of the plates and profiles after rolling, marking, cutting and bending as per the structural design drawings or models, followed by erection and launching. Other joining techniques are used for other materials like fibre reinforced plastic and glass-reinforced plastic.
Science and craft

Traditionally, naval architecture has been more craft than science. The suitability of a vessel's shape was judged by looking at a half-model of a vessel or a prototype. Ungainly shapes or abrupt transitions were frowned on as being flawed. This included, rigging, deck arrangements, and even fixtures. Subjective descriptors such as ungainly, full, and fine were used as a substitute for the more precise terms used today. A vessel was, and still is described as having a ‘fair’ shape. The term ‘fair’ is meant to denote not only a smooth transition from fore to aft but also a shape that was ‘right.’ Determining what is ‘right’ in a particular situation in the absence of definitive supporting analysis encompasses the art of naval architecture to this day.
Modern low-cost digital computers and dedicated software, combined with extensive research to correlate full-scale, towing tank and computational data, have enabled naval architects to more accurately predict the performance of a marine vehicle. These tools are used for static stability (intact and damaged), dynamic stability, resistance, powering, hull development, structural analysis, green water modelling, and slamming analysis. Data is regularly shared in international conferences sponsored by RINA, Society of Naval Architects and Marine Engineers (SNAME) and others. Computational Fluid Dynamics is being applied to predict the response of a floating body in a random sea.

Due to the complexity associated with operating in a marine environment, naval architecture is a co-operative effort between groups of technically skilled individuals who are specialists in particular fields, often coordinated by a lead naval architect.[5] This inherent complexity also means that the analytical tools available are much less evolved than those for designing aircraft, cars and even spacecraft. This is due primarily to the paucity of data on the environment the marine vehicle is required to work in and the complexity of the interaction of waves and wind on a marine structure.
A naval architect is an engineer who is responsible for the design, construction, and/or repair of ships, boats, other marine vessels, and offshore structures, both commercial and military, including:
- Merchant ships - oil tankers, gas tankers, cargo ships, bulk carriers, container ships
- Passenger/vehicle ferries, cruise ships
- Warships - frigates, destroyers, aircraft carriers, amphibious ships
- Submarines and underwater vehicles
- Icebreakers
- High speed craft - hovercraft, multi-hull ships, hydrofoil craft
- Workboats - barges, fishing boats, anchor handling tug supply vessels, platform supply vessels, tug boats, pilot vessels, rescue craft
- Yachts, power boats, and other recreational watercraft
- Offshore platforms and subsea developments
Some of these vessels are amongst the largest such as supertankers and most complex such as Aircraft carriers and highly valued movable structures produced by mankind. They are the most efficient method of transporting the world's raw materials and products known to man. Modern engineering on this scale is essentially a team activity conducted by specialists in their respective fields and disciplines. Naval architects integrate these activities. This demanding leadership role requires managerial qualities and the ability to bring together the often-conflicting demands of the various design constraints to produce a product which is fit for the purpose.
In addition to this leadership role, a naval architect also has a specialist function in ensuring that a safe, economic, and seaworthy design is produced. To undertake all these tasks, a naval architect must have an understanding of many branches of engineering and must be in the forefront of high technology areas. He or she must be able to effectively utilize the services provided by scientists, lawyers, accountants, and business people of many kinds.
Naval architects typically work for shipyards, ship owners, design firms and consultancies, equipment manufacturers, Classification societies, regulatory bodies (Admiralty law), navies, and governments.
Marine engineering :

A view of a ship's engine room
Marine propulsion is the mechanism or system used to generate thrust to move a ship or boat across water. While paddles and sails are still used on some smaller boats, most modern ships are propelled by mechanical systems consisting a motor or engine turning a propeller, or less frequently, in jet drives, an impeller. Marine engineering is the discipline concerned with the design of marine propulsion systems.
Steam engines were the first mechanical engines used in marine propulsion, but have mostly been replaced by two-stroke or four-stroke diesel engines, outboard motors, and gas turbine engines on faster ships. Nuclear reactors producing steam are used to propel warships and icebreakers, and there have been attempts to utilize them to power commercial vessels. Electric motors have been used on submarines and electric boats and have been proposed for energy-efficient propulsion.
Power sources
Pre-mechanisation

A wind propelled fishing boat in Mozambique
Until the application of the steam engine to ships in the early 19th century, oars propelled galleys, or the wind propelled sailing ships. Before mechanisation, merchant ships always used sail, but as long as naval warfare depended on ships closing to ram or to fight hand-to-hand, galleys dominated in marine conflicts because of their maneuverability and speed. The Greek navies that fought in the Peloponnesian War used triremes, as did the Romans at the Battle of Actium. The use of large numbers of cannon from the 16th century meant that maneuverability took second place to broadside weight; this led to the dominance of the sail-powered warship.
In modern times, human propulsion is found mainly on small boats or as auxiliary propulsion on sailboats. Human propulsion includes the pole, still widely used in marshy areas, rowing which was used even on large galleys, and the pedals.
Propulsion by sail generally consists of a sail hoisted on an erect mast, supported by stays and spars and controlled by ropes. Sail systems were the dominant form of propulsion until the nineteenth century. They are now generally used for recreation and racing, although experimental sail systems, such as the kites/royals, turbosails, rotorsails, wingsails, windmills and SkySails's own kite buoy-system have been used on larger modern vessels for fuel savings.
Reciprocating steam engines
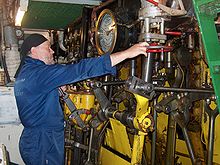
Main article: Marine steam engine
The development of piston-engined steamships was a complex process. Early steamships were fueled by wood, later ones by coal or fuel oil. Early ships used stern or side paddle wheels, while later ones used screw propellers.
The first commercial success accrued to Robert Fulton's North River Steamboat (often called Clermont) in the US in 1807, followed in Europe by the 45-foot Comet of 1812. Steam propulsion progressed considerably over the rest of the 19th century. Notable developments included the steam surface condenser, which eliminated the use of sea water in the ship's boilers. This permitted higher steam pressures, and thus the use of higher efficiency multiple expansion (compound) engines. As the means of transmitting the engine's power, paddle wheels gave way to more efficient screw propellers.
Steam turbines
Steam turbines were fueled by coal or, later, fuel oil or nuclear power. The marine steam turbine developed by Sir Charles Algernon Parsons raised the power-to-weight ratio. He achieved publicity by demonstrating it unofficially in the 100-foot Turbinia at the Spithead Naval Review in 1897. This facilitated a generation of high-speed liners in the first half of the 20th century, and rendered the reciprocating steam engine obsolete; first in warships, and later in merchant vessels.
In the early 20th century, heavy fuel oil came into more general use and began to replace coal as the fuel of choice in steamships. Its great advantages were convenience, reduced manpower by removal of the need for trimmers and stokers, and reduced space needed for fuel bunkers.
In the second half of the 20th century, rising fuel costs almost led to the demise of the steam turbine. Most new ships since around 1960 have been built with diesel engines. The last major passenger ship built with steam turbines was the Fairsky, launched in 1984. Similarly, many steam ships were re-engined to improve fuel efficiency. One high profile example was the 1968 built Queen Elizabeth 2 which had her steam turbines replaced with a diesel-electric propulsion plant in 1986.
Most new-build ships with steam turbines are specialist vessels such as nuclear-powered vessels, and certain merchant vessels (notably Liquefied Natural Gas (LNG) and coal carriers) where the cargo can be used as bunker fuel.
LNG carriers
New LNG carriers (a high growth area of shipping) continue to be built with steam turbines. The natural gas is stored in a liquid state in cryogenic vessels aboard these ships, and a small amount of 'boil off' gas is needed to maintain the pressure and temperature inside the vessels within operating limits. The 'boil off' gas provides the fuel for the ship's boilers, which provide steam for the turbines, the simplest way to deal with the gas. Technology to operate internal combustion engines (modified marine two-stroke diesel engines) on this gas has improved, however, so such engines are starting to appear in LNG carriers; with their greater thermal efficiency, less gas is burnt. Developments have also been made in the process of re-liquefying 'boil off' gas, letting it be returned to the cryogenic tanks. The financial returns on LNG are potentially greater than the cost of the marine-grade fuel oil burnt in conventional diesel engines, so the re-liquefaction process is starting to be used on diesel engine propelled LNG carriers. Another factor driving the change from turbines to diesel engines for LNG carriers is the shortage of steam turbine qualified seagoing engineers. With the lack of turbine powered ships in other shipping sectors, and the rapid rise in size of the worldwide LNG fleet, not enough have been trained to meet the demand. It may be that the days are numbered for marine steam turbine propulsion systems, even though all but sixteen of the orders for new LNG carriers at the end of 2004 were for steam turbine propelled ships.

The NS Savannah was the first nuclear-powered cargo-passenger ship
Nuclear-powered steam turbines
In these vessels, the nuclear reactor heats water to create steam to drive the turbines. Due to low prices of diesel oil, nuclear propulsion is rare except in some Navy and specialist vessels such as icebreakers. In large aircraft carriers, the space formerly used for ship's bunkerage could be used instead to bunker aviation fuel. In submarines, the ability to run submerged at high speed and in relative quiet for long periods holds obvious advantages. A few cruisers have also employed nuclear power; as of 2006, the only ones remaining in service are the Russian Kirov class. An example of a non-military ship with nuclear marine propulsion is the Arktika class icebreaker with 75,000 shaft horsepower (55,930 kW). Commercial experiments such as the NS Savannah have so far proved uneconomical compared with conventional propulsion.
In recent times, there is some renewed interest in commercial nuclear shipping. Nuclear powered cargo ships could lower costs associated with carbon dioxide emissions and travel at higher cruise speeds than conventional diesel powered vessels.
Reciprocating diesel engines
A modern diesel engine aboard a cargo ship
Most modern ships utilise a reciprocating diesel engine as their prime mover, due to their operating simplicity, robustness and fuel economy compared to most other prime mover mechanisms. The rotating crankshaft can be directly coupled to the propeller with slow speed engines, via a reduction gearbox for medium and high speed engines, or via an alternator and electric motor in diesel-electric vessels.
The reciprocating marine diesel engine first came into use in 1903 when the diesel electric rivertanker Vandal was put in service by Branobel. Diesel engines soon offered greater efficiency than the steam turbine, but for many years had an inferior power-to-space ratio. The advent of turbocharging however hastened their adoption, by permitting greater power densities.
Diesel engines today are broadly classified according to
- Their operating cycle: two-stroke engine or four-stroke engine
- Their construction: crosshead, trunk, or opposed piston
- Their speed
- Slow speed: any engine with a maximum operating speed up to 300 revolutions per minute (rpm), although most large two-stroke slow speed diesel engines operate below 120 rpm. Some very long stroke engines have a maximum speed of around 80 rpm. The largest, most powerful engines in the world are slow speed, two stroke, crosshead diesels.
- Medium speed: any engine with a maximum operating speed in the range 300-900 rpm. Many modern four-stroke medium speed diesel engines have a maximum operating speed of around 500 rpm.
- High speed: any engine with a maximum operating speed above 900 rpm.
Most modern larger merchant ships use either slow speed, two stroke, crosshead engines, or medium speed, four stroke, trunk engines. Some smaller vessels may use high speed diesel engines.
The size of the different types of engines is an important factor in selecting what will be installed in a new ship. Slow speed two-stroke engines are much taller, but the footprint required, is smaller than that needed for equivalently rated four-stroke medium speed diesel engines. As space above the waterline is at a premium in passenger ships and ferries (especially ones with a car deck), these ships tend to use multiple medium speed engines resulting in a longer, lower engine room than that needed for two-stroke diesel engines. Multiple engine installations also give redundancy in the event of mechanical failure of one or more engines, and the potential for greater efficiency over a wider range of operating conditions.
As modern ships' propellers are at their most efficient at the operating speed of most slow speed diesel engines, ships with these engines do not generally need gearboxes. Usually such propulsion systems consist of either one or two propeller shafts each with its own direct drive engine. Ships propelled by medium or high speed diesel engines may have one or two (sometimes more) propellers, commonly with one or more engines driving each propeller shaft through a gearbox. Where more than one engine is geared to a single shaft, each engine will most likely drive through a clutch, allowing engines not being used to be disconnected from the gearbox while others keep running. This arrangement lets maintenance be carried out while under way, even far from port.
Gas turbines
Combined marine propulsion |
---|
CODOG CODAG CODLAG CODAD COSAG COGOG COGAG COGAS CONAS |
Many warships built since the 1960s have used gas turbines for propulsion, as have a few passenger ships, like the jetfoil. Gas turbines are commonly used in combination with other types of engine. Most recently, the Queen Mary 2 has had gas turbines installed in addition to diesel engines. Because of their poor thermal efficiency at low power (cruising) output, it is common for ships using them to have diesel engines for cruising, with gas turbines reserved for when higher speeds are needed however, in the case of passenger ships the main reason for installing gas turbines has been to allow a reduction of emissions in sensitive environmental areas or while in port. Some warships, and a few modern cruise ships have also used the steam turbines to improve the efficiency of their gas turbines in a combined cycle, where waste heat from a gas turbine exhaust is utilized to boil water and create steam for driving a steam turbine. In such combined cycles, thermal efficiency can be the same or slightly greater than that of diesel engines alone; however, the grade of fuel needed for these gas turbines is far more costly than that needed for the diesel engines, so the running costs are still higher.
Screws
Propeller#Marine
The technical term for what is often described as a "propeller" on a ship is a screw. The differentiation is necessary as the two systems, although similar in appearance, have very different physical properties. There are many variations of marine screw systems, including twin, contra-rotating, controllable-pitch, and nozzle-style screws. Smaller vessels tend to have a single screw. Some modern aircraft carriers use four propellers, supplemented with bow and stern-thrusters. Power is transmitted from the engine to the screw by way of a propeller shaft, which may or may not be connected to a gearbox.
Paddle wheels

Left: original paddle wheel from a paddle steamer.
Right: detail of a paddle steamer.
See also: Paddle steamer and Pedalo
The paddle wheel is a large wheel, generally built of a steel framework, upon the outer edge of which are fitted numerous paddle blades (called floats or buckets). The bottom quarter or so of the wheel travels underwater. Rotation of the paddle wheel produces thrust, forward or backward as required. More advanced paddle wheel designs have featured feathering methods that keep each paddle blade oriented closer to vertical while it is in the water; this increases efficiency. The upper part of a paddle wheel is normally enclosed in a paddlebox to minimise splashing.
Petroleum engineering :
Petroleum engineering is an engineering discipline concerned with the activities related to the production of hydrocarbons, which can be either crude oil or natural gas. Subsurface activities are deemed to fall within the upstream sector of the oil and gas industry, which are the activities of finding and producing hydrocarbons. Refining and distribution to a market are referred to as the downstream sector. Exploration, by earth scientists, and petroleum engineering are the oil and gas industry's two main subsurface disciplines, which focus on maximizing economic recovery of hydrocarbons from subsurface reservoirs. Petroleum geology and geophysics focus on provision of a static description of the hydrocarbon reservoir rock, while petroleum engineering focuses on estimation of the recoverable volume of this resource using a detailed understanding of the physical behavior of oil, water and gas within porous rock at very high pressure.
The combined efforts of geologists and petroleum engineers throughout the life of a hydrocarbon accumulation determine the way in which a reservoir is developed and depleted, and usually they have the highest impact on field economics. Petroleum engineering requires a good knowledge of many other related disciplines, such as geophysics, petroleum geology, formation evaluation (well logging), drilling, economics, reservoir simulation, well engineering, artificial lift systems, and oil and gas facilities engineering.
Overview
Petroleum engineering has become a technical profession that involves extracting oil in increasingly difficult situations as much of the "low hanging fruit" of the world's oil fields has been found and depleted. Improvements in computer modeling, materials and the application of statistics, probability analysis, and new technologies like horizontal drilling and enhanced oil recovery, have drastically improved the toolbox of the petroleum engineer in recent decades.
Deep-water, arctic and desert conditions are commonly contended with. High Temperature and High Pressure (HTHP) environments have become increasingly commonplace in operations and require the petroleum engineer to be savvy in topics as wide ranging as thermo-hydraulics, geomechanics, and intelligent systems.
The Society of Petroleum Engineers (SPE) is the largest professional society for petroleum engineers and publishes much information concerning the industry. Petroleum engineering education is available at 17 universities in the United States and many more throughout the world - primarily in oil producing regions - and some oil companies have considerable in-house petroleum engineering training classes.
Petroleum engineering has historically been one of the highest paid engineering disciplines, although there is a tendency for mass layoffs when oil prices decline. In a June 4th, 2007 article, Forbes.com reported that petroleum engineering was the 24th best paying job in the United States. The 2010 National Association of Colleges and Employers survey showed petroleum engineers as the highest paid 2010 graduates at an average $125,220 annual salary. For individuals with experience, salaries can go from $170,000 to $260,000 annually.
Types
Petroleum engineers divide themselves into several types:
- Reservoir engineers work to optimize production of oil and gas via proper well placement, production rates, and enhanced oil recovery techniques.
- Drilling engineers manage the technical aspects of drilling exploratory, production and injection wells.
- Production engineers, including subsurface engineers, manage the interface between the reservoir and the well, including perforations, sand control, downhole flow control, and downhole monitoring equipment; evaluate artificial lift methods; and also select surface equipment that separates the produced fluids (oil, natural gas, and water).
Other courses
- STCW Courses
- GMDSS Schedule and other details
- Short Course for Sailing Marine Engineers
STCW :
International Convention on Standards of Training, Certification and Watchkeeping for Seafarers | ||
---|---|---|
Signed | 7 July 1978 | |
Location | London, U.K. | |
Effective | 28 April 1984 | |
Condition | 25 ratifications, the combined merchant fleets of which constitute not less than 50% of the gross tonnage of the world's merchant shipping of ships of 100 gross tonnage or more | |
Parties | 155 | |
Depositary | Secretary-General of the Inter-Governmental Maritime Consultative Organization (IMCO) | |
Languages | English, French, Russian and Spanish |
The International Convention on Standards of Training, Certification and Watchkeeping for Seafarers (or STCW), 1978 sets qualification standards for masters, officers and watch personnel on seagoing merchant ships. STCW was adopted in 1978 by conference at the International Maritime Organization (IMO) in London, and entered into force in 1984. The Convention was significantly amended in 1995.
The 1978 STCW Convention was the first to establish basic requirements on training, certification and watchkeeping for seafarers on an international level. Previously the standards of training, certification and watchkeeping of officers and ratings were established by individual governments, usually without reference to practices in other countries. As a result standards and procedures varied widely, even though shipping is the most international of all industries.
The Convention prescribes minimum standards relating to training, certification and watchkeeping for seafarers which countries are obliged to meet or exceed.
The Convention did not deal with manning levels: IMO provisions in this area are covered by regulation 14 of Chapter V of the International Convention for the Safety of Life at Sea (SOLAS), 1974, whose requirements are backed up by resolution A.890(21) Principles of safe manning, adopted by the IMO Assembly in 1999, which replaced an earlier resolution A.481(XII) adopted in 1981.
One especially important feature of the Convention is that it applies to ships of non-party States when visiting ports of States which are Parties to the Convention. Article X requires Parties to apply the control measures to ships of all flags to the extent necessary to ensure that no more favourable treatment is given to ships entitled to fly the flag of a State which is not a Party than is given to ships entitled to fly the flag of a State that is a Party.
The difficulties which could arise for ships of States which are not Parties to the Convention is one reason why the Convention has received such wide acceptance. By 2011, the STCW Convention had 155 Parties, representing 98.9 percent of world shipping tonnage.
1995 revision
On July 7, 1995 the IMO adopted a comprehensive revision of STCW. They also included a proposal to develop a new STCW Code, which would contain the technical details associated with provisions of the Convention. The amendments entered force on February 1, 1997. Full implementation was required by February 1, 2002. Mariners already holding licenses had the option to renew those licenses in accordance with the old rules of the 1978 Convention during the period ending on February 1, 2002. Mariners entering training programs after August 1, 1998 are required to meet the competency standards of the new 1995 Amendments.
The most significant amendments concerned:
- a) enhancement of port state control;
- b) communication of information to IMO to allow for mutual oversight and consistency in application of standards,
- c) quality standards systems (QSS), oversight of training, assessment, and certification procedures,
- The Amendments require that seafarers be provided with "familiarization training" and "basic safety training" which includes basic fire fighting, elementary first aid, personal survival techniques, and personal safety and social responsibility. This training is intended to ensure that seafarers are aware of the hazards of working on a vessel and can respond appropriately in an emergency.
- d) placement of responsibility on parties, including those issuing licenses, and flag states employing foreign nationals, to ensure seafarers meet objective standards of competence, and
- e) rest period requirements for watchkeeping personnel.
GMDSS :
The Global Maritime Distress and Safety System (GMDSS) is an internationally agreed-upon set of safety procedures, types of equipment, and communication protocols used to increase safety and make it easier to rescue distressed ships, boats and aircraft.
GMDSS consists of several systems, some of which are new, but many of which have been in operation for many years. The system is intended to perform the following functions: alerting (including position determination of the unit in distress), search and rescue coordination, locating (homing), maritime safety information broadcasts, general communications, and bridge-to-bridge communications. Specific radio carriage requirements depend upon the ship's area of operation, rather than its tonnage. The system also provides redundant means of distress alerting, and emergency sources of power.
Recreational vessels do not need to comply with GMDSS radio carriage requirements, but will increasingly use the Digital Selective Calling (DSC) VHF radios. Offshore vessels may elect to equip themselves further. Vessels under 300 Gross tonnage (GT) are not subject to GMDSS requirements.
Components of GMDSS
The main types of equipment used in GMDSS are:
Emergency Position-Indicating Radio Beacon (EPIRB)
Emergency Position-Indicating Radio Beacon :
Cospas-Sarsat is an international satellite-based search and rescue system, established by Canada, France, the United States, and Russia. These four countries jointly helped develop the 406 MHz Emergency Position-Indicating Radio Beacon (EPIRB), an element of the GMDSS designed to operate with Cospas-Sarsat system. These automatic-activating EPIRBs, now required on SOLAS ships, commercial fishing vessels, and all passenger ships, are designed to transmit to alert rescue coordination centers via the satellite system from anywhere in the world. The original COSPAS / SARSAT system used Polar orbiting satellites but in recent years the system has been expanded to also include 4 geostationary satellites. Newest designs incorporate GPS receivers to transmit highly accurate positions (within about 20 metres)of the distress position. The original COSPAS / SARSAT satellites could calculate EPIRB position to within about 3 nautical miles (5.6 km) by using Doppler techniques. By the end of 2010 EPIRB manufacturers may be offering AIS (Automatic Identification System) enabled beacons. The service-ability of these items are checked monthly and annually and have limited battery shelf life between 2 to 5 years using mostly Lithium type batteries. 406 MHz EPIRB's transmit a registration number which is linked to a database of information about the vessel.
NAVTEX
Navtex is an international, automated system for instantly distributing maritime safety information (MSI) which includes navigational warnings, weather forecasts and weather warnings, search and rescue notices and similar information to ships. A small, low-cost and self-contained "smart" printing radio receiver is installed on the bridge, or the place from where the ship is navigated, and checks each incoming message to see if it has been received during an earlier transmission, or if it is of a category of no interest to the ship's master. The frequency of transmission of these messages is 518 kHz in English, while 490 kHz is sometime used to broadcast in a local language. The messages are coded with a header code identified by the using single letters of the alphabet to represent broadcasting stations, type of messages, and followed by two figures indicating the serial number of the message. For example: FA56 where F is the ID of the transmitting station, A indicates the message category Navigational warning, and 56 is the consecutive message number.
Inmarsat
Satellite systems operated by the Inmarsat, overseen by IMSO, International Mobile Satellite Organization are also important elements of the GMDSS. The types of Inmarsat ship earth station terminals recognized by the GMDSS are: Inmarsat B, C and F77. Inmarsat B and F77, an updated version of the now redundant Inmarsat A, provide ship/shore, ship/ship and shore/ship telephone, telex and high-speed data services, including a distress priority telephone and telex service to and from rescue coordination centers. Fleet 77 fully supports the Global Maritime Distress and Safety System (GMDSS) and includes advanced features such as emergency call prioritisation. The Inmarsat C provides ship/shore, shore/ship and ship/ship store-and-forward data and email messaging, the capability for sending preformatted distress messages to a rescue coordination center, and the Inmarsat C SafetyNET service. The Inmarsat C SafetyNET service is a satellite-based worldwide maritime safety information broadcast service of high seas weather warnings, NAVAREA navigational warnings, radionavigation warnings, ice reports and warnings generated by the USCG-conducted International Ice Patrol, and other similar information not provided by NAVTEX. SafetyNET works similarly to NAVTEX in areas outside NAVTEX coverage.
Inmarsat C equipment is relatively small and lightweight, and costs much less than an Inmarsat B or F77. Inmarsat B and F77 ship earth stations which require relatively large gyro-stabilized uni directional antennas; the antenna size of the Inmarsat C is much smaller and is omni directional.
Under a cooperative agreement with the National Oceanic and Atmospheric Administration (NOAA), combined meteorological observations and AMVER reports can now be sent to both the USCG AMVER Center, and NOAA, using an Inmarsat C ship earth station, at no charge. .
SOLAS now requires that Inmarsat C equipment have an integral satellite navigation receiver, or be externally connected to a satellite navigation receiver. That connection will ensure accurate location information to be sent to a rescue coordination center if a distress alert is ever transmitted.
Also the new LRIT long range tracking systems are upgraded via GMDSS Inmarsat C which are also compliant along with inbuilt SSAS, or Ship Security Alert System. SSAS provides a means to covertly transmit a security alert distress message to local authorities in the event of a mutiny, pirate attack, or other hostile action towards the vessel or its crew.
High Frequency
A GMDSS system may include High Frequency (HF) radiotelephone and radiotelex (narrow-band direct printing) equipment, with calls initiated by digital selective calling (DSC). Worldwide broadcasts of maritime safety information can also made on HF narrow-band direct printing channels.
Search and Rescue Locating device
Search and Rescue Transponder :
The GMDSS installation on ships include one (two on vessels over 500 GT) Search and Rescue Locating device(s) called Search and Rescue Radar Transponders (SART) which are used to locate survival craft or distressed vessels by creating a series of twelve dots on a rescuing ship's 3 cm radar display. The detection range between these devices and ships, dependent upon the height of the ship's radar mast and the height of the Search and Rescue Locating device, is normally about 15 km (8 nautical miles). Once detected by radar, the Search and Rescue Locating device will produce a visual and aural indication to the persons in distress.
Digital Selective Calling
Digital Selective Calling :
The IMO also introduced Digital Selective Calling (DSC) on MF, HF and VHF maritime radios as part of the GMDSS system. DSC is primarily intended to initiate ship-to-ship, ship-to-shore and shore-to-ship radiotelephone and MF/HF radiotelex calls. DSC calls can also be made to individual stations, groups of stations, or "all stations" in one's radio range. Each DSC-equipped ship, shore station and group is assigned a unique 9-digit Maritime Mobile Service Identity.
DSC distress alerts, which consist of a preformatted distress message, are used to initiate emergency communications with ships and rescue coordination centers. DSC was intended to eliminate the need for persons on a ship's bridge or on shore to continuously guard radio receivers on voice radio channels, including VHF channel 16 (156.8 MHz) and 2182 kHz now used for distress, safety and calling. A listening watch aboard GMDSS-equipped ships on 2182 kHz ended on February 1, 1999. In May 2002, IMO decided to postpone cessation of a VHF listening watch aboard ships. That watchkeeping requirement had been scheduled to end on 1 February 2005.
IMO and ITU both require that the DSC-equipped MF/HF and VHF radios be externally connected to a satellite navigation receiver (GPS). That connection will ensure accurate location information is sent to a rescue coordination center if a distress alert is transmitted. The FCC requires that all new VHF and MF/HF maritime radiotelephones type accepted after June 1999 have at least a basic DSC capability.
VHF digital selective calling also has other capabilities beyond those required for the GMDSS. The Coast Guard uses this system to track vessels in Prince William Sound, Alaska, Vessel Traffic Service. IMO and the USCG also plan to require ships carry a Universal Shipborne Automatic Identification System, which will be DSC-compatible. Countries having a GMDSS A1 Area should be able to identify and track AIS-equipped vessels in its waters without any additional radio equipment. A DSC-equipped radio cannot be interrogated and tracked unless that option was included by the manufacturer, and unless the user configures it to allow tracking.
GMDSS telecommunications equipment should not be reserved for emergency use only. The International Maritime Organization encourages mariners to use GMDSS equipment for routine as well as safety telecommunications.
GMDSS sea areas
GMDSS sea areas serve two purposes: to describe areas where GMDSS services are available, and to define what radio equipment GMDSS ships must carry (carriage requirements). Prior to the GMDSS, the number and type of radio safety equipment ships had to carry depended upon its tonnage. With GMDSS, the number and type of radio safety equipment ships have to carry depends upon the GMDSS areas in which they travel.
In addition to equipment listed below, all GMDSS-regulated ships must carry a satellite EPIRB, a NAVTEX receiver (if they travel in any areas served by NAVTEX), an Inmarsat-C SafetyNET receiver (if they travel in any areas not served by NAVTEX), a DSC-equipped VHF radiotelephone, two (if between 300 and less than 500 GRT) or three VHF handhelds (if 500 GRT or more), and two 9 GHz search and rescue radar transponders (SART).
Sea Area A1
An area within the radiotelephone coverage of at least one VHF coast station in which continuous digital selective calling (Ch.70/156.525 MHz) alerting and radiotelephony services are available.Such an area could extend typically 30 nautical miles (56 km) to 40 nautical miles (74 km) from the Coast Station.
Sea Area A2
An area, excluding Sea Area A1, within the radiotelephone coverage of at least one MF coast station in which continuous DSC (2187.5 kHz) alerting and radiotelephony services are available.For planning purposes this area typically extends to up to 180 nautical miles (330 km) offshore during daylight hours,but would exclude any A1 designated areas.In practice,satisfactory coverage may often be achieved out to around 400 nautical miles (740 km) offshore during night time...
Sea Area A3
An area,excluding sea areas A1 and A2, within the coverage of an INMARSAT geostationary satellite. This area lies between about latitude 76 Degree NORTH and SOUTH,but excludes A1 and/or A2 designated areas.Inmarsat guarantee their system will work between 70 South and 70 North though it will often work to 76 degrees South or North.
Sea Area A4
An area outside Sea Areas A1, A2 and A3 is called Sea Area A4. This is essentially the polar regions, north and south of about 76 degrees of latitude, excluding any A1 or A2 areas.
GMDSS radio equipment required for U.S. coastal voyages
Presently, until an A1 or A2 Sea Area is established, GMDSS-mandated ships operating off the U.S. coast must fit to Sea Areas A3 (or A4) regardless of where they operate. U.S. ships whose voyage allows them to always remain within VHF channel 16 coverage of U.S. Coast Guard stations may apply to the Federal Communications Commission for an individual waiver to fit to Sea Area A1 requirements. Similarly, those who remain within 2182 kHz coverage of U.S. Coast Guard stations may apply for a waiver to fit to Sea Area A2 requirements.
History of GMDSS
Since the invention of radio at the end of the 19th century, ships at sea have relied on Morse code, invented by Samuel Morse and first used in 1844, for distress and safety telecommunications. The need for ship and coast radio stations to have and use radiotelegraph equipment, and to listen to a common radio frequency for Morse encoded distress calls, was recognized after the sinking of the liner RMS Titanic in the North Atlantic in 1912. The U.S. Congress enacted legislation soon after, requiring U.S. ships to use Morse code radiotelegraph equipment for distress calls. The International Telecommunications Union (ITU), now a United Nations agency, followed suit for ships of all nations. Morse encoded distress calling has saved thousands of lives since its inception almost a century ago, but its use requires skilled radio operators spending many hours listening to the radio distress frequency. Its range on the medium frequency (MF) distress band (500 kHz) is limited, and the amount of traffic Morse signals can carry is also limited.
Not all ship-to-shore radio communications were short range. Some radio stations provided long-range radiotelephony services, such as radio telegrams and radio telex calls, on the HF bands (3-30 MHz) enabling worldwide communications with ships. For example, Portishead Radio, which was the world's busiest radiotelephony station, provided HF long-range services. In 1974, it had 154 radio operators who handled over 20 million words per year. Such large radiotelephony stations employed large numbers of people and were expensive to operate. By the end of the 1980s, satellite services had started to take an increasingly large share of the market for ship-to-shore communications.
For these reasons, the International Maritime Organization (IMO), a United Nations agency specializing in safety of shipping and preventing ships from polluting the seas, began looking at ways of improving maritime distress and safety communications. In 1979, a group of experts drafted the International Convention on Maritime Search and Rescue, which called for development of a global search and rescue plan. This group also passed a resolution calling for development by IMO of a Global Maritime Distress and Safety System (GMDSS) to provide the communication support needed to implement the search and rescue plan. This new system, which the world's maritime nations are implementing, is based upon a combination of satellite and terrestrial radio services, and has changed international distress communications from being primarily ship-to-ship based to ship-to-shore (Rescue Coordination Center) based. It spelled the end of Morse code communications for all but a few users, such as amateur radio operators. The GMDSS provides for automatic distress alerting and locating in cases where a radio operator doesn't have time to send an SOS or MAYDAY call, and, for the first time, requires ships to receive broadcasts of maritime safety information which could prevent a distress from happening in the first place. In 1988, IMO amended the Safety of Life at Sea (SOLAS) Convention, requiring ships subject to it fit GMDSS equipment. Such ships were required to carry NAVTEX and satellite EPIRBs by 1 August 1993, and had to fit all other GMDSS equipment by 1 February 1999. US ships were allowed to fit GMDSS in lieu of Morse telegraphy equipment by the Telecommunications Act of 1996.
Licensing of operators
National maritime authorities may issue various classes of licenses. The General Operator’s Certificate is required on SOLAS vessels operating also outside GMDSS Sea Area A1, while a Restricted Operator’s Certificate is needed on SOLAS vessels operated solely within GMDSS Sea Area A1,
Long Range Certificate may be issued, and is required on non-SOLAS vessels operating outside GMDSS Sea Area A1, while a Short Range Certificate is issued for non-SOLAS vessels operating only inside GMDSS Sea Area A1.
Finally there is a Restricted radiotelephone operator's certificate, which is similar to the Short Range Certificate but limited VHR DSC radio operation. Some countries do not consider this adequate for GMDSS qualification.
In the United States four different GMDSS certificates are issued. A GMDSS Radio Maintainer's License allows a person to maintain, install,and repair GMDSS equipment at sea. A GMDSS Radio Operator's License is necessary for a person to use required GMDSS equipment. The holder of both certificates can be issued one GMDSS Radio Operator/Maintainer License. Finally, the GMDSS Restricted License is available for VHF operations only within 20 nautical miles (37 km) of the coast. To obtain any of these licenses a person must be a U.S. citizen or otherwise eligible for work in the country, be able to communicate in English, and take written examinations approved by the Federal Communications Commission. Like the amateur radio examinations, these are given by private, FCC-approved groups. These are generally not the same agencies who administer the ham tests. Written test elements 1 and 7 are required for the Operator license, and elements 1 and 7R for the Restricted Operator. (Passing element 1 also automatically qualifies the applicant for the Marine Radiotelephone Operator Permit, the MROP.)
For the Maintainer license, written exam element 9 must be passed. However, to obtain this certificate an applicant must also hold a General radiotelephone operator license (GROL), which requires passing commercial written exam elements 1 and 3 (and thus supersedes the MROP). Upon the further passing of optional written exam element 8 the ship radar endorsement will be added to both the GROL and Maintainer licenses. This allows the holder to adjust, maintain, and repair shipboard radar equipment.
Until March 25, 2008 GMDSS operator and maintainer licenses expired after five years but could be renewed upon payment of a fee. On that date all new certificates were issued valid for the lifetimes of their holders. For those still valid but previously issued with expiration dates, the FCC states:
Any GMDSS Radio Operator's License, Restricted GMDSS Radio Operator's License, GMDSS Radio Maintainer's License, GMDSS Radio Operator/Maintainer License, or Marine Radio Operator Permit that was active, i.e., had not expired, as of March 25, 2008, does not have to be renewed.
Since an older certificate does show an expiration date, for crewmembers sailing internationally it may be worth paying the fee (as of 2010 it was $60) to avoid any confusion with local authorities.
Finally, to actually serve as a GMDSS operator on most commercial vessels the United States Coast Guard requires additional classroom training and practical experience beyond just holding a license.
Awards
- Best Training Award of The Lloyd's List (Asian section)
- Best maritime academy award (at the 2nd South East CEO Conclave & Awards 2010)
- Sailor Today Award for "Innovation in Maritime Training" in 2008.
- CRISIL as graded all DG Approved courses as Grade I – Excellent
National/International collaborations
AMET has got collaborations with many universities and academies in India and abroad.AMET is conducting HND programs in collaboration with south tyneside college and Glasgow college of nautical sciences.Dept of Naval architecture has got collaboration with universities of Glasgow and Strathclyde .Amet is also conducting two B.sc(Hons) degrees in collaboration with University of Plymouth.The university also has Tie-up with IIT Madras,DNV Academy India, AP-mollar Denmark
Photo of University
My cousin recommended this blog and she was totally right keep up the fantastic work!
ReplyDeleteNautical Science Course in India